New 3D Lung Model Points Away From Tissue Stiffening as Key to Fibrosis
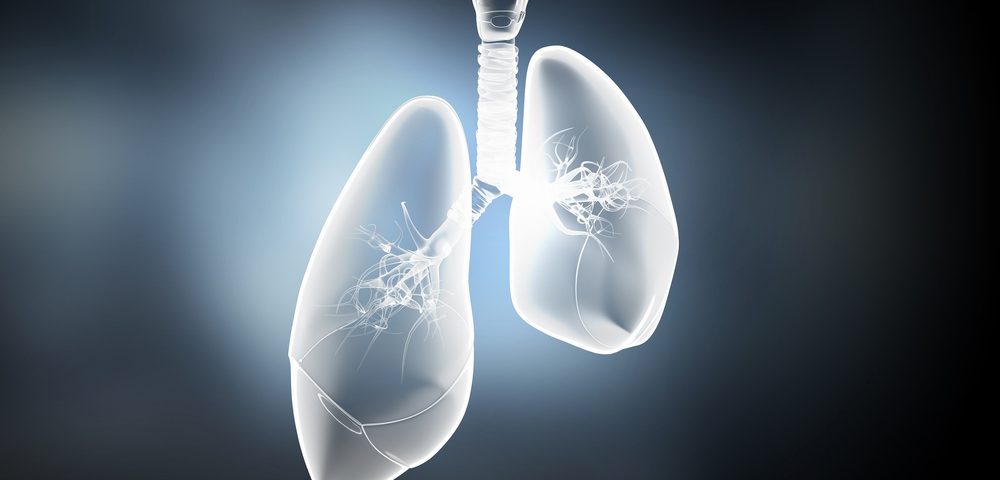
A new 3D model of lung tissue allowed the scientists who developed it to identify aspects of pulmonary fibrosis (PF) not evident in the 2D models often used in research.
This new model, developed by a research team at the University of Michigan, revealed potentially important aspects of fibrosis and its progression not seen in the earlier models. Namely, it showed that high fiber density, rather than tissue stiffening, affected the behavior of cells in ways similar to what happens in patients’ lung tissue, the researchers stated.
This 3D model can help in the study of fibrosis and in better identifying antifibrotic therapies, some 90% of which show promise in animal work but “fail to translate to clinical settings,” the team added.
The study describing the model, “Microengineered 3D pulmonary interstitial mimetics highlight a critical role for matrix degradation in myofibroblast differentiation” was published in the journal Science Advances.
PF is characterized by scarring (fibrosis) of lung tissue, which leads to tissue stiffening in alveoli — the tiny air sacs in the lungs responsible for gas exchange — and ultimately to difficulties in breathing.
Its causes are not fully understood, but fibroblasts — cells found in a type of lung tissue called interstitium — are known to play a key role in fibrotic processes.
After an injury, lung tissue sends mechanical signals that activate fibroblasts, converting them into myofibroblasts. Paradoxically, if myofibroblasts are properly controlled, they play a critical role in wound healing; when they get out of control, they produce an excess of proteins in the lungs that ultimately result in scar tissue.
Research today is focused on treatments better able to relieve disease symptoms or slow its progression. But the effective of therapies are difficult to replicate in 2D conditions, where cells are grown on flat plastic materials such as Petri dishes or on hydrogel surfaces, the researchers wrote.
In fact, 2D models often fail to recreate what happens in the human body to accurately spot those therapeutic compounds most likely to help patients.
Previous “models use patient-derived cells that are affordable, scalable, and amenable to microscopy,” the researchers wrote, “but often fail to recapitulate the complex 3D matrix structure of the interstitial tissue regions where fibrotic diseases … originate.”
To address this limitation, researchers at the University of Michigan developed a new 3D tissue engineered model of fibrotic lung tissue.
The team constructed a multicomponent hydrogel representing a 3D lung interstitium — the tissue where fibroblasts are located and fibrosis begins. Their goal was to understand how mechanical signals from lung tissue influence fibroblast behavior and PF progression.
“Our lung tissue model looks and behaves similarly to what we have observed when imaging real lung tissue,” Brendon Baker, PhD, the study’s lead author and an assistant professor at Michigan, said in a university press release.
According to the team, research in PF has been focused on targeting the considerable lung stiffening seen in patients. But these researchers believe that targeting stiffness alone is not enough to stop disease progression in patients, even if it works in cells cultivated in dish models.
They first analyzed how tissue stiffening leads to the production of myofibroblasts in both a 2D and their 3D model.
Results differed between these models. Myofibroblast production was inversely correlated with hydrogel stiffness in the 3D model — meaning that greater stiffness resulted in lesser myofibroblasts and thus lesser scar tissue — while greater stiffness in 2D models resulted in increased myofibroblast production and activation.
“Even in cells from the same patient, we saw different outcomes,” said Daniel Matera, the study’s first author. “When we introduced stiffness into the 2D testing environment, it activated myofibroblasts, essentially creating scar tissue.
“When we introduced that same kind of stiffness into our 3D testing environment, it prevented or slowed the activation of myofibroblasts, stopping or slowing the creation of scar tissue,” Matera added.
Researchers then investigated whether changes in fiber density, reflecting fibrosis-associated alterations, could influence myofibroblasts in their 3D model.
They found that greater fiber density promoted myofibroblast production and activation in this model.
“Heightened fiber density promotes a fibrotic phenotype … despite the absence of a stiff surrounding hydrogel,” the researchers wrote.
These “findings demonstrate a clear influence of fiber density on [myofibroblast] differentiation and phenotype in 3D and furthermore suggest that this in vitro model recapitulates key pathogenic events associated with the progression of fibrosis in vivo,” they added.
Using bioinformatics, researchers also saw that the activity of matrix metalloproteinases — proteins responsible for the degradation of most extracellular matrix proteins during growth and normal tissue turnover — is essential for myofibroblast activation in 3D, but not in more simplified 2D, settings.
To further underscore differences between this 3D model and earlier models, the team tested several antifibrotic agents — including Esbriet (pirfenidone, marketed by Genentech) and Ofev (nintedanib, by Boehringer Ingelheim), approved therapies for idiopathic pulmonary fibrosis (IPF).
The antifibrotic effects of these two therapies were evident in the 3D model, but not in 2D tissue culture models traditionally used for compound screening.
“Recreating the 3D fibrous structure of the lung interstitium allowed us to confirm effective drugs that wouldn’t be identified as hits in traditional screening settings,” Baker said. “Patient cells within our model can actively stiffen, degrade or remodel their own environment just like they do in disease.”
Future work on this model may allow it to serve as a more reliable platform for testing potential PF treatments, the researchers wrote.
Overall, these results “demonstrate a departure from the established relationship between stiffness and myofibroblast differentiation in 2D, and provide a new 3D model for studying fibrosis and identifying antifibrotic therapeutics,” the team concluded.