Model of Pulmonary Fibrosis Details Gene Changes at Specific Disease Stages, Study Reports
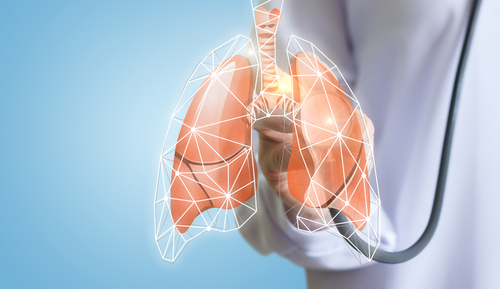
A model of the human lung constructed with the help of tissue samples from 10 idiopathic pulmonary fibrosis (IPF) patients details changes in gene activity that precede evident fibrosis, a study reports.
This model is a sort of genetic roadmap to IPF progression that may help researchers find treatments for specific stages of IPF.
The study “Transcriptional regulatory model of fibrosis progression in the human lung” was published in the Journal of Clinical Investigation Insight.
Fibrotic progression in IPF, which leads to chronic and damaging scaring of the lung, can be treated with two U.S. Food and Drug Administration (FDA)-approved therapies, Ofev (nintedanib) by Boehringer Ingelheim, and Esbriet (pirfenidone) from Genentech.
“The drugs may not be pleasant, but they work,” Naftali Kaminski, MD, the Boehringer-Ingelheim endowed professor of internal medicine at Yale School of Medicine, said in a university news story. “There’s hope on the horizon.”
However, neither of these therapies are able to reverse the extensive fibrosis in the lungs of IPF patients.
This is partly because it is still not clear what molecular changes occur in IPF that change normal lung architecture to that seen in advanced IPF. According to the researchers, this information could be the key to developing better treatments.
“My group has felt for years that to develop interventions for IPF that are more effective, we need to understand how the disease progresses in the human lung,” Kaminski said.
The researchers developed a new human model to study IPF. They used 95 lung samples to do so, from 10 IPF patients and six people with healthy lungs serving as control samples.
To understand which genes are important in IPF progression, researchers classified the samples according to their tissue architecture: controls, IPF with no fibrosis, IPF with moderate fibrosis, and IPF with advanced fibrosis. For this, they used standard techniques to assess tissue architecture, and a technique called microCT, an X-ray imaging in 3D.
The team also quantified the activity of genes (gene expression) by measuring the changes in RNA molecules and microRNAs. (Of note, the information in a gene, its DNA, is transcribed into a RNA molecule that, in turn, can be translated into a protein. Micro RNAs are small RNA molecules that do not code for a protein, but they regulate the expression of genes.)
With this analysis, researchers were able to identify a set of genes that changed before fibrosis was evident in tissue. Results suggest that normal tissue from IPF lungs were already showing abnormal genetic alterations, and that depending on the disease stage (moderate progressive or advanced fibrosis) genes were differently regulated.
“This is the first evidence that different stages of the disease may require different interventions,” Kaminski said.
In particular, a gene called POU2AF1 is involved in fibrosis according to the model and showed increased activity in immune cells, called B-lymphocytes, located in the IPF lungs. When the team deleted this gene in a mouse model of IPF (the bleomycin-induced mouse model), they observed that mice kept a normal lung structure and were protected from fibrotic development.
“Our results suggest that different regulatory mechanisms are active in minimally affected, progressively affected, and end-stage tissues in IPF,” researchers wrote.
Moreover, this suggests that abnormal signaling pathways in IPF may be also common in other diseases, opening the possibility of success in IPF therapy development aiding other disorders.
“What I would like to do now is accelerate translation. To make this data publicly available for drug repurposing,” said Kaminski.
The new model is available as an interactive website that can be used by other researchers.